 |
Information box |
The main purpose of this site is to extend the
intraoperative monitoring to include the neurophysiologic
parameters with intraoperative navigation guided with Skyra 3
tesla MRI and other radiologic facilities to merge the
morphologic and histochemical data in concordance with the
functional data.
CNS Clinic
Located in Jordan Amman near Al-Shmaisani hospital, where all
ambulatory activity is going on.
Contact: Tel: +96265677695, +96265677694.
Skyra running
A magnetom Skyra 3 tesla MRI with all clinical applications
started to run in our hospital in 28-October-2013.
Shmaisani hospital
The hospital where the project is located and running diagnostic
and surgical activity. |
|
 |
|
 |
Electroencephalography is the
technique by which the electrical activity generated by the
brain is amplified and displayed, resulting in an
electroencephalogram (EEG). This method enables one to assess
brain function noninvasively over a given period. Although many
abnormalities on the EEG are nonspecific, several clinical
presentations have associated EEG findings that are diagnostic
of a specific condition or lesion in the central nervous
system. Before the advent of modern neuroimaging, the EEG was
one of the most important noninvasive diagnostic tools available
to the neurologist and neurosurgeon. It provided information on
cerebral function when anatomic detail could not be accurately
obtained. Current neuroimaging techniques such as computed
tomography (CT) and magnetic resonance imaging (MRI) of the
brain now yield excellent neuroanatomic detail. Despite these
advances, the EEG remains a valuable tool in the clinical
evaluation of many disorders of the central nervous system, as
it is readily available and safe and provides information on
brain function that is still unique.
When an EEG
is requested, it is important that the referring physician state
the clinical question that is to be answered by the EEG. Common
reasons for obtaining an EEG include a history of a clinical
seizure and the need to rule out epileptiform activity; acute
encephalopathy or coma of undetermined etiology; or a prolonged
seizure with the need to rule out ongoing electrographic seizure
activity (i.e., status epilepticus). When the EEG is completed,
the findings are summarized in a report using accepted EEG
terminology, with the most significant findings listed first.
The EEG is also interpreted in the context of the clinical
presentation and question, thus providing the clinician with a
clinical correlation to the findings noted in the EEG.
 |
History |
 |
The early
evaluations of the central nervous system by physiologists in
the late 1700s and early 1800s consisted of stimulating the
brain electrically rather than measuring the electrical currents
it generates. Not until the latter half of the nineteenth
century did the British physiologist Richard Caton describe the
electrical activity of the brain in experimental animals. Caton
obtained cortical EEG recordings, and he also noted that'
'feeble currents of varying direction pass through the
multiplier when the electrodes are placed on two points of the
external surface of the skull.
Early in the twentieth century, the Russian
physiologist V.V. PravdichNeminsky used the term "electrocerebrogram,"
and he defined the predominant frequency
bands of the cerebral electrical activity in animals, labeling
them alpha and beta. In 1929 Hans Berger published the initial
findings on the EEG in humans, calling it the "Elektrenkephalogramm",
from which
electroencephalogram has been derived. Previous investigators
had noted the reactivity of the EEG in animals to peripheral
somatic electrical stimulation. Berger showed that the human EEG
is reactive to opening and closing of the eyes: such potential
changes from the occipital region were later termed the Berger,
or alpha, rhythm.
In 1934, Berger's findings
were confirmed by Adrian and Matthews. The application of EEG
in a neuropathologic condition was initially described by Walter
when he demonstrated focal EEG slowing in patients with brain
tumors, which he called delta waves. During the subsequent
two decades, clinical investigators evaluated the use of the EEG
in normal and neuropathologic conditions. Over the past six
decades. standards have also been developed for the application
and nomenclature of electrode placement and montage
representation. The clinical significance of most EEG patterns
has been well described. Advances in electronics and computers
have been applied to electroencephalography, providing improved
definition of both cerebral and extracerebral activity (such as
artifacts). The EEG now is
"paperless," with a digitized EEG displayed in real time on a
video monitor. Frequency spectral analysis (brain mapping) is
being actively investigated, and it proved to be an
additional tool in the evaluation of brain function.
 |
Technical
Aspects |
 |
The electrical activity of the
brain has an amplitude in the microvolt range, typically
ranging from 10 to 150 µV. In a routine EEG, the brain's
electrical activity is measured at the scalp using a surface
electrode. The electrical signal is then conducted by wire to
the EEG machine, where it is amplified, filtered, and displayed.
This process is briefly summarized below.
 |
Electrodes |
 |
Since the
discovery of the EEG. several types of electrodes have been
used. Subdermal needle electrodes were the first to be applied.
However, owing to the variability of impedance and the potential
for morbidity and transmission of infectious disease, this type
of electrode is no longer routinely used. The most common
electrode currently in use is a gold-plated disc 10 mm in
diameter. Twenty-one electrode sites on the scalp are defined
according to the International 10-20 System, which is based
on skull landmarks (inion, nasion and left and right preauricular points) whose distances are then subdivided in a
specified manner. A typical interelectrode distance is 6 cm.
Scalp electrodes are identified by a letter and number (Fig-1). Most of the letters specify an approximate brain region.
as follows: Fp: frontopolar; F: frontal;
C: central; T: temporal; P:
parietal; and O: occipital. The ear electrode is denoted by the
letter A. Electrodes with odd numbers are on the left side of
the head (Fp1, F3, C3, P3, O1, F7, T3, T5, and A1), and
electrodes with even numbers are on the right side (Fp2, F4, C4,
P4, 02, F8, T4, T6, and A2). Midline electrodes are designated
by the letter "z"
(Fpz, Fz, Cz, Pz, and Oz).
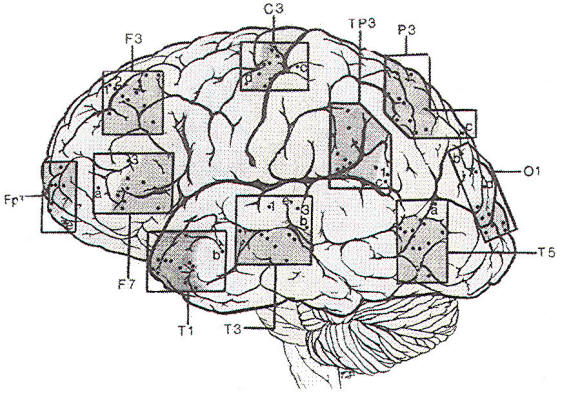 |
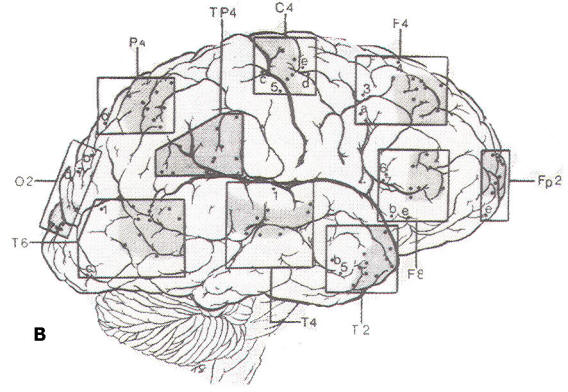 |
Fig-1: |
After marking the scalp
according to the International 10-20 System, the technologist
prepares each site by using a mild abrasive to lower and
equalize the scalp impedance. An electrode is placed at each
site using either a conductive paste or a collodionsoaked gauze
patch through which conductive gel is injected into the disc.
Properly prepared electrodes have impedances between 1000 and
5000 Ω.
Scalp electrodes provide
adequate measurement of the cerebral electrical activity arising
from the superior and lateral aspects of the brain. The
anterolateral temporal lobe can be sampled by using a pair of
"true" temporal electrodes (T1 and T2), in addition to the
10-20 System electrodes. However, the midline and basal aspects
of the brain cannot be sampled well by electrodes on the scalp.
In the past the nasopharyngeal electrode was used in an attempt
to measure the electrocerebral activity of the anteromesial
aspect of the temporal lobe. It consisted of a silver rod that
was advanced through the naris until it came in contact with the
posterior wall of the nasopharynx. However, it was subject to
significant artifacts caused by breathing and swallowing. The
sphenoidal electrode is an alternative that can be used to
semiinvasively sample the anteromesial temporal lobe. It
consists of a thin, Teflon-coated platinum or chlorided silver
wire that is placed near the foramen ovale. Using sterile
technique, the sphenoidal electrode is inserted with a 20 or 22
gauge spinal needle, 1cm anterior to the tragus, beneath the zygomatic arch and toward the foramen
ovale, approximately 3 to 4
cm deep to the skin.
Invasive
monitoring of cortical electrical activity is performed using
depth electrodes or subdural strip or grid array electrodes. The
depth electrode is a thin, flexible Teflon sheath having 6 or 8
concentric stainless steel or platinum contacts along it with
interelectrode distances of 5 or 10 mm. It is placed
stereotactically using a rigid introducer, which is removed
after electrode placement Subdural strip or grid array
electrodes consist of stainless steel or platinum discs embedded
in a Silastic or Teflon sheet The electrode contacts are
separated by distances typically measuring 1 cm. Subdural
electrodes are placed through a craniotomy site, the size of
which is determined by the size of the electrode strip or array.
These invasive electrodes may be used either extraoperatively
during video-EEG monitoring or intraoperatively during surgical
excision. Their primary use is to more accurately define an
epileptic focus. In addition, the cortical surface electrodes
can be used to stimulate the surface of the brain to determine
the function of a specific area of cortex, such as speech,
language comprehension, or motor control.
 |
EEG Machine |
 |
The
cerebral electrical activity is conducted by wires from the scalp
and/or invasive electrodes to the jackbox of the EEG machine.
The inputs to the jackbox are then used to compose a montage,
which is a specific arrangement or array of electrodes that
display the EEG. The EEG machines currently available use 16,
18, or 21 channels. Each channel consists of a differential
amplifier, which compares the input of two electrodes and
amplifies the output to the pen-writing system or video display
screen.
An upward pen deflection is defined as negative, and
occurs when input I is negative with respect to input 2 or when
input 2 is positive with respect to input I. A downward pen
deflection is defined as positive, and occurs when input 1 is
positive with respect to input 2 or when input 2 is negative
with respect to input 1. These two conditions are illustrated in
Fig-2, where there is a downward deflection in channel 1
because input 2 is more negative than input 1, and an upward
deflection in channel 2 because input 1 is more negative than
input 2. This example demonstrates a surfacenegative phase
reversal in a bipolar montage, which localizes maximal surface electronegativity. Depending on the polarity of each input, or
electrode, there may be summation, no change, or cancellation
of the cerebral EEG activity between the two inputs.
Cancellation indicates a region of isoelectricity, and the
result is no change in the output of the amplifier and no pen
deflection. Before
being displayed, the amplifier outputs are filtered, typically
using a low-frequency filter setting of I Hz and a highfrequency filter setting of 70 Hz. A 60-Hz "notch" filter
may be used in a recording environment with excessive electrical
interference, such as an intensive care unit. The amplitude of
each channel can be adjusted by changing the
amplifier gain, or sensitivity. The amplified,
filtered outputs are then displayed either with an
analog pen writing system or digitally on a video
monitor. The routine paper speed is 30 cm/s, so that
each page of EEG displays 10 s of electrical
cerebral activity. A paper speed of I5 cm/s is often used in neonatal EEGs in order to
compress the EEG and accentuate focal slowing. A faster paper
speed such as 60 cm/sec can be used to expand the time scale in
an attempt to see if two potentials or events occur
synchronously or to better define high-frequency activity. |
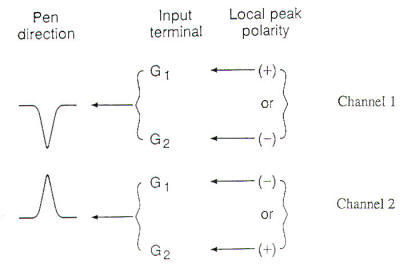 |
Fig-2:Pen deflection based on
inputs G1 and G2 into channels 1 and 2. |
 |
Montages |
 |
The brain's electrical
activity is logically displayed using different montages, which
are specific arrangements of electrodes. Each montage provides
the electroencephalographer with a different view, to delineate
both normal and abnormal activity. The objective of any montage
is to display the electrical field potentials generated by
cortical neurons. The output from each channel in a montage
represents the voltage difference of the inputs from each pair
of electrodes into the differential amplifier.
Current standards specify that
each montage attempt to maintain a linear arrangement of
electrodes having equal interelectrode distances. The display is
oriented from anterior to posterior and from left to right. A
bipolar montage is constructed by linking successive electrodes
into sequential channels. In a referential montage, each
electrode is 'referred' to a reference electrode, such as the
ipsilateral ear or the vertex (Cz). The most commonly used
montages are the longitudinal (anterior to posterior, or AP)
bipolar montage, the transverse (left to right) bipolar montage,
and the referential (to the ipsilateral ear) montage.
Typically, bipolar montages
are used to localize the region of an abnormality, This is often
seen as a phase reversal between two or more electrodes in a
given region. Referential montages are useful to define the
field or distribution of the abnormality by the amplitude of
electrocerebral activity at the electrodes in the region of
interest. Both types of montage have their disadvantages,
Bipolar montages are susceptible to field cancellation because
adjacent electrodes may be isoelectric in potential. Also, if
the region of interest lies at the end of the linear chain of
electrodes, no phase reversal will be apparent. In referential
montages, it is important to be aware that if the reference is
located in the field of the cerebral electrical signal of
interest (called an active
reference),
cancellation or reverse polarity may be seen in
the channels to which uninvolved electrodes are referenced.
Finding an uninvolved or inactive reference may be difficult.
 |
Obtaining the
EEG |
 |
Including the patient setup
time, a routine EEG takes approximately 60 to 90 min and
produces a 30-min recording. Electroencephalography on patients
in the intensive care unit (lCU) or on neonates often takes
longer, both because setting up takes longer and because a
longer recording is made. The ICU is often a hostile environment
for electroencephalography, owing to the abundance of electrical
monitoring equipment, which may result in an excessive 60-Hz
noise artifact on the EEG recording,
While placing the electrodes, the technologist
obtains the patient's clinical history and past medical history,
and a family history for epilepsy or clinical problems similar
to those of the patient. Medications currently being taken are
listed, especially ones that may affect the EEG, such as
barbiturates, benzodiazepines, tricyclic antidepressants, or neuroleptic
medications. Medication for sedation or sleep induction is also
noted. If there is a skull defect from previous trauma or
intracranial surgery, it is depicted diagrammatically on the
front sheet of the EEG. At the beginning of the EEG recording,
electrical and biological calibrations are performed. The
sensitivity, high-frequency filter, time constant, or
low-frequency filter, and the use of any other special filters
(e.g., a 60-Hz notch filter) are also noted on the first page of
each montage, as well as the level of consciousness and the
mental state of the patient. Approximately 10 min of
uninterrupted recording are performed for each montage.
Longitudinal bipolar, transverse bipolar, and referential
montages are obtained, and the technologist may also obtain
additional montages to better display a suspected abnormality.
The patient is allowed to fall asleep, and, later in the
recording, attempts are made to fully alert the patient by
testing memory or calculations. Also, during the recording, photic stimulation is performed to evaluate for photosensitive
seizures. Last, the patient is asked to hyperventilate for 3 to
5 min in an attempt to accentuate focal slowing or focal or
generalized epileptiform activity.
 |
EEG
Terminology |
 |
A standard terminology is used
to consistently describe each EEG. These terms summarize the
electrocerebral activity as well as any abnormal waveform or
transient in each region of the brain during the EEG. These
terms are frequency, amplitude, polarity, morphology,
distribution, rhythmicity, synchrony, reactivity, and
persistence. Each term will be briefly discussed below.
Frequency refers to the
repetition rate or number of cycles per second (Hz) of a given
waveform. The frequency of a single waveform can be calculated
from the inverse of the peak-to-peak duration of the waveform
(1/time). During periods when the EEG is relatively sinusoidal,
the frequency can be estimated by counting the number of cycles
per 1 second epoch. Four frequency bands appear in EEGs and have
been named delta (0.5 to 3.5 Hz), theta (4.0 to 7.5 Hz), alpha
(8.0 to 12.5 Hz), and beta (13 Hz and greater).
Amplitude is the magnitude of
the EEG activity in microvolts (µV).
It is determined by measuring
the pen deflection in millimeters (mm) at a specified machine
sensitivity (µV /mm). Most EEGs are performed at a
sensitivity of 7
µV /mm, such that a 10
mm pen deflection
signifies an amplitude of 70
µV. In describing the EEG,
quantitative measures may be used (i.e., 50 to 70
µV), or a
qualitative scale may be used, in which low amplitude is
defined as less than 20
µV, medium amplitude as 25 to 95
µV,
and high amplitude as greater than 100
µV.
Polarity is the sign of the
EEG activity and may be negative, positive, or isoelectric
(i.e., zero). By convention, upward pen deflection signifies
negative polarity, and downward pen deflection signifies
positive polarity.
Morphology refers to the shape
of the EEG waveform. It may be regular (i.e., sinusoidal) or
irregular, monophasic, or polyphasic (e.g., a triphasic wave).
The morphology of a transient is essential to determining
whether the transient is normal or abnormal, nonepileptiform or
epileptiform.
Distribution of EEG
activity may be focal or generalized. If focal, the activity
should be defined by side and region involved (i.e., frontal,
temporal, central, parietal, occipital, or midline). Generalized
activity is widespread, involving both hemispheres equally.
Although widespread, generalized activity is often either
anteriorly or posteriorly predominant.
Rhythmicity: The EEG is
rhythmic when it has a sinusoidal pattern at a relatively
constant frequency. Arrhythmic activity is a mix of frequencies
and morphologies.
Synchrony: EEG activity that occurs at
the same time in different regions of the brain is called
synchronous. Activity that occurs at the same time and same
location on both sides of the scalp is bilaterally synchronous.
or bisynchronous. Conversely, activity that occurs at different
times is asynchronous.
Reactivity refers to
alteration in the EEG activity caused by stimulation of the
patient. This is accomplished by visual stimulation (opening
and closing the eyes), noxious stimulation (pinching the
patient), auditory stimulation (a loud noise), or cognitive
stimulation (simple arithmetic calculations). An unreactive EEG
is one that shows no variation in activity over all scalp leads
despite vigorous attempts at stimulation.
Persistence: A specific EEG activity
appearing in a given region of the brain can be either
intermittent or persistent. A persistent activity is present in
the region for at least 70 to 80 percent of the record, despite
stimulation and state change. EEG activity that is present in
the region for less than 70 to 80 percent of the record is
called intermittent, and may be further designated as rare,
occasional, or
frequent,
depending on its total amount in the record.
 |
Normal EEG |
 |
The age of the patient and the
level of consciousness (i.e., awake or asleep) are critical
parameters in describing the normal EEG, as both factors
determine the frequency, amplitude, polarity, morphology,
distribution, rhythmicity, synchrony, reactivity and
persistence of the activities that are recorded. The EEG of the
neonate is significantly different from that of the infant of 3
months or older, and it will be discussed below.
In the
normal awake EEG, the most notable feature is a posteriorly
dominant, rhythmic activity that is symmetric, bisynchronous,
and reactive. This activity has been called the alpha rhythm.
which must be distinguished from the previously described alpha
frequency range of 8 to 12.5 Hz. At approximately 3 months of
age, a posteriorly dominant background alpha rhythm can be seen,
which is high-amplitude, 3 to 4-Hz activity. From infancy
through the early teenage years. the mean frequency of the
background alpha rhythm increases gradually to 10 Hz. and the
amplitude decreases to moderate voltage (Fig-3). These
values then persist throughout adulthood and old age. Subharmonics (one-half normal frequency) and harmonics
(twice normal frequency) of the alpha rhythm occur in a small
percentage of normal individuals. These variants are reactive to
various stimuli. A pattern of lowamplitude, mixed fast
frequencies can also be seen in normal adults, which may be due
in part to subjects' inability to relax adequately during the
EEG. In the central head regions of adult subjects, the EEG
consists of moderate to low-amplitude alpha and theta-range
frequencies. whereas in the frontal head regions. lowamplitude
beta-range frequencies are generally seen. These activities
should be bisynchronous and symmetric. In 10 to 20 percent of
young adults, either or both central head regions may show
rhythmic, arciform alpha-frequency activity that is reactive to
the patient performing mental arithmetic operations or moving
the contralateral hand. This is called mu rhythm. |
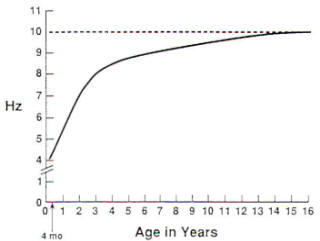 |
Fig-3: Background alpha rhythm and
age relation. |
Sleep has been divided into
non-rapid-eye movement (NREM) and rapid-eye-movement (REM)
phases. NREM has four stages: stage I (drowsy), stage II, stage
III and stage IV. As the patient becomes drowsy, the background
alpha rhythm becomes arrhythmic, with intermixed theta and beta
frequencies that spread into the central head regions. A slow
lateral eye movement artifact may be visualized on the EEG in
the anterolateral head regions, because the retina is
electronegative with respect to the cornea, resulting in an
electrical dipole whose field changes with eye movement. Two
additional features of stage I sleep are sharply contoured, surfacepositive theta transients of moderate amplitude that
appear synchronously or asynchronously in the posterior head
regions (positive occipital sharp transients of sleep or POSTS)
and moderate to high-amplitude, sharply contoured, biphasic
theta or alpha transients that phase-reverse at the vertex
(vertex sharp waves). Stage II of sleep is defined by the
presence of K complexes and sleep spindles. The K complex is a
high-amplitude. biphasic slow wave of 0.5 to I s duration that
has a distribution similar to that of the vertex sharp wave. The
sleep spindle consists of rhythmic. moderate-amplitude alpha
frequency activity lasting 0.5 to 1 s which is bisynchronous in
the central head regions. In deeper stage I and stage II sleep,
the remaining background consists of moderate to low-amplitude
mixed theta, alpha, and beta frequencies. In stages III and IV
of sleep, there is increasing delta activity having high
amplitude and anterior predominance. In REM sleep, the EEG
consists of diffusely distributed, moderate to low-amplitude
mixed frequencies with rapid eye movement artifacts seen in the
anterolateral head regions. The features of NREM sleep are
absent during REM sleep (i.e., vertex sharp waves, sleep
spindles, and K complexes).
 |
Benign
Variants and Artifacts |
 |
One of the major goals of EEG
is to accurately define which EEG patterns are consistent with
the diagnosis of seizures, and which patterns may be of no
clinical significance (that is, normal). The "epileptiform"
patterns and "seizure-like" discharges that are not
significantly associated with seizures are called benign EEG variants and, in general, are considered normal findings on
the EEG when it is properly obtained. For each of these
patterns, the interpretation depends critically on the age and
clinical state of the patient and the distribution, frequency,
amplitude, and morphology of the waveform(s). The benign
epileptiform patterns include benign epileptiform transients of
sleep (BETS), 14 and 6-Hz positive bursts, 6-Hz spike and wave
(phantom spike and
wave),
and wicket spikes. The benign
seizure-like discharges include rhythmic midtemporal
discharges (RMTD or psychomotor variant), midline theta
rhythm, frontal arousal rhythm (FAR), and subclinical
rhythmic electrographic discharges in adults (SREDA).
An EEG activity that does
not originate from the brain is called an artifact.
Artifacts can be divided into two major groups, physiologic
and nonphysiologic. The accurate identification of artifacts
can be crucial to the correct interpretation of both normal
and abnormal EEGs. An electrically hostile environment such
as an ICU often proves to be a significant challenge to the
EEG technologist, who must recognize and, if possible,
eliminate all artifacts. Any source in the body that has an
electrical dipole or generates an electrical field is
capable of producing a physiologic artifact. These include
the heart (electrocardiogram and ballistocardiogram or pulse
artifact), eyes (oculomotor artifact), muscles (myogenic
artifact), and tongue (glossokinetic artifact). Sweating may
alter the impedance at the electrode-scalp interface and
produce an artifact. In the region of a skull defect, there
may be accentuation of amplitude with very sharp morphology,
which is called breach rhythm.
Examples of nonphysiologic artifacts include
60-Hz interference from nearby electrical equipment, kinesiogenic artifacts caused by patient or electrode
movement, IV drip artifact caused by a charged saline
solution, and mechanical ventilator artifacts caused by
patient movement or fluid movement in the ventilator tubing.
 |
Abnormal EEG |
 |
Most abnormal EEG findings
are defined by localizing the region of maximal electrode
negativity associated with the abnormality. Models of
radially oriented neurons have been proposed to define the
origin of cortical electronegativity. However, much of the
brain's cortical surface lies along the base and walls of
sulci. Using scalp and cortical electrodes, Cooper and
colleagues estimated that approximately 6 cm2 of cortical
surface was necessary to generate scalp-recorded electrical
potentials. Abraham and AjmoneMarsan have demonstrated
that only 20 to 70 percent of spike discharges seen using
electrocorticography are seen on scalp EEG. Significant
abnormalities on the EEG consist of slowing, lack of
reactivity, interictal epileptiform activity. periodic
patterns, and ictal patterns. The clinical and pathologic
importance of each finding depends on whether it is focal or
generalized, intermittent or persistent. Although an
amplitude asymmetry of greater than 50 percent is also
considered abnormal, it must be demonstrated on multiple
montages, including a referential montage, preferably to a
common reference. Amplitude asymmetries are often the result
of normal anatomic variations (e.g., in skull thickness) or
technical factors (interelectrode distances. electrode
impedances. etc.).
As noted
above. the normal frequency range for the background alpha
rhythm is from 8 to 12.5 Hz. Therefore, in a maximally alerted
adult patient, a background alpha rhythm of less than 8 Hz is
considered abnormal. Intermittent, generalized delta slowing
may appear as isolated diffuse polymorphic delta transients or
as rhythmic delta activity. Intermittent rhythmic delta activity
(IRDA) may be seen having frontal predominance (FIRDA) in adults
or occipital predominance (OIRDA) in children. These findings
are nonspecific in etiology. However, each abnormality noted
above is consistent with diffuse bihemispheric cerebral
dysfunction. In adult patients, the severity of the cerebral
dysfunction is related to the degree of theta or delta slowing
of the posterior background frequencies or to
the total amount of generalized delta slowing that occurs during
the EEG record.
Persistent frequency
asymmetries of greater than 1 Hz between corresponding scalp
regions are abnormal. Focal slow transients in the delta range
often have variable morphology (are polymorphic) and are
considered abnormal in all fully alerted, adult patients, with
the exception of rare dominant-hemisphere temporal delta slowing
in the elderly. When focal delta slowing is present for 70 to 80
percent of the record, it is called persistent polymorphic
delta activity, or PPDA. Although not specific in etiology,
focal PPDA is consistent with a structural lesion in the absence
of a recent transient neurologic event such as a seizure,
transient ischemic attack, or complicated migraine headache. If
focal polymorphic delta activity appears in less than 70
percent of the record, it is noted as intermittent and qualified
as rare, occasional, or frequent, depending on the total amount
seen during the EEG. Intermittent focal delta slowing is
nonspecific in etiology and clinical significance and is thought
to be consistent with focal neuronal or cerebral dysfunction in
the region of the slowing.
Generalized loss of reactivity
is evidence of severe diffuse bihemispheric cerebral
dysfunction, regardless of the dominant frequency. This finding
is commonly seen in coma and will be discussed in more detail
below. Focal loss of reactivity may be seen in
the setting of an intracerebral structural abnormality, such as
a cerebral infarct, abscess, or tumor. Focal unreactivity of the
posterior background rhythm with eye opening is called Bancaud's
phenomenon, because of its location, it is the most readily
recognized form of focal unreactivity.
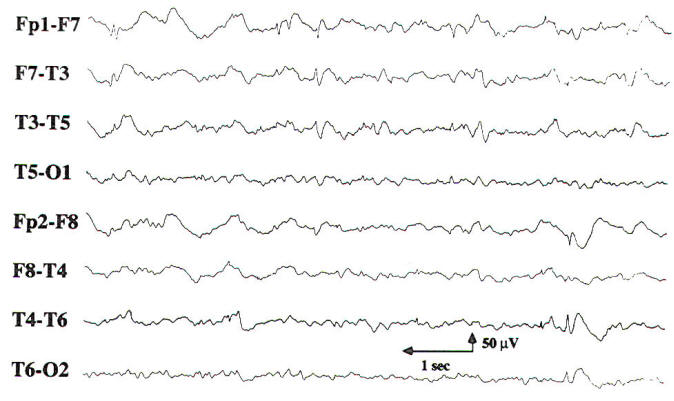 |
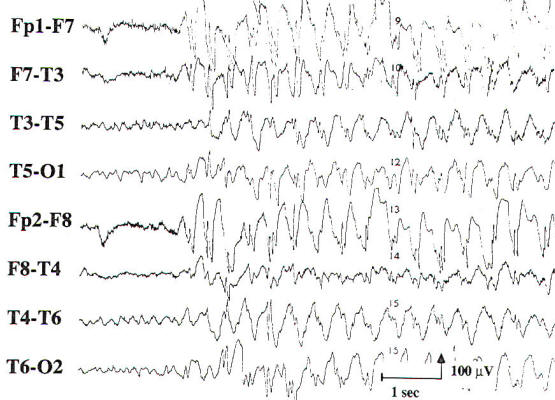 |
Fig-4: Bilateral independent
temporal spikes and sharp waves. Left side greater
than right. |
Fig-5: Burst of generalized 3-Hz
spike and wave activity. |
Two transients of special
interest are the sharp wave and the spike discharge. These
transients are important because of their high correlation with
seizures, and they are often referred to as epileptiform
discharges. They are defined by their morphology and duration,
with sharp waves having a duration of 70 to 200 ms and spike
discharges having a duration of 20 to 70 ms. When accompanied
by an after-going slow wave, they are referred to as a sharp or
spike and slow wave complex. If an epileptiform discharge
appears focally, it is localized by finding a region with a
phase reversal on the bipolar montages (Fig-4). Using a
referential montage with an uninvolved reference. a focal epileptiform discharge is localized by defining the region of
greatest electronegativity. The generalized epileptiform
transient is most commonly a spike discharge, which may appear
as an isolated spike, a spike-wave complex, or a polyspike and
wave complex. Although they are called generalized, these
discharges are often anteriorly predominant and may have
shifting left or right sided emphasis, which averages out during
the EEG. Spike-wave and polyspike-wave complexes often appear as
repetitive discharges having a predominant frequency based on
the repetition rate of the discharges (Fig-5 ).
The clinical significance of
the epileptiform discharge will be discussed in more detail
later. It must be properly recognized and
distinguished from benign variants, artifacts, and normal EEG
activity. Focal delta slowing in the same region as a suspected epileptiform discharge is additional evidence for focal neuronal
dysfunction in the region of the presumed epileptic focus.
Sharp waves and spikes
generally occur intermittently. In certain clinical settings,
such as acute hemispheric cerebral infarction, sharp waves
appear in a periodic fashion, and they are then referred to as
periodic lateralized epileptiform discharges (PLEDs). PLEDs are
most often seen in acute structural brain lesions. Generalized
periodic sharp wave activity is classified on the basis of its
morphology and frequency along with the specific clinical
presentation. Examples include triphasic waves, generalized
periodic epileptiform discharges (GPEDs), and the burst
suppression pattern. Each of these patterns indicates that there
is severe diffuse cerebral dysfunction.
An epileptic seizure rarely
occurs during an EEG. The hallmark features of an ictal pattern
are an evolution in frequency and field of the EEG activity
during the event. Evolution in frequency refers to an increase or
decrease from the initial frequency: evolution in field refers
to spread of the activity into adjoining regions. The amplitude
of the activity may increase, decrease, or remain the same
during the ictal pattern or discharge. When there is an
accompanying change in the clinical state of the patient, these
findings are diagnostic of a seizure disorder. Ictal patterns
that are not accompanied by a clinical change in the patient are
called subclinical seizures. If the ictal discharge is focal in
onset, then the seizure disorder is said to be partial in
origin. However, if the discharge is generalized at onset, the
seizure disorder may be either generalized or partial in origin,
as a focal midline seizure focus may project equally to both
hemispheres with a wide field. The morphology of the discharges,
age of the patient, and ictal semiology are important factors
in defining the type of seizure.
Activation
procedures are used to enhance or increase abnormalities on the EEG.
Although focal slowing is the abnormality most likely to be
"activated," these procedures may also accentuate epileptiform
activity or induce a seizure. Activation procedures currently in
use consist of hyperventilation (HV), intermittent photic
stimulation (IPS), spontaneous and medicationinduced sleep, and
sleep after sleep deprivation. In the past, injections of
seizure-inducing drugs such as pentylenetetrazol were used
during the EEG to activate spike foci and induce seizures.
However, these techniques are no longer used owing to the risk
to the patient and the difficulty of discriminating spontaneous
from drug-induced interictal and ictal discharges.
Hyperventilation should be
performed for 3 to 5 min by any cooperative patient at least
once during the EEG, provided there are no medical
contraindications (cardiopulmonary disease, unstable cerebrovascular disease, etc.). Focal delta slowing that has
been noted during wakefulness or drowsiness is often accentuated
during hyperventilation. The induction of typical 3-Hz
generalized spike and wave discharges and absence seizures by
hyperventilation is well known. Hyperventilation has also been
found to activate focal epileptiform discharges much less often
than generalized epileptiform discharges. If hyperventilation
provokes an absence seizure in a patient with an idiopathic
generalized epilepsy, clinical unresponsiveness should be
confirmed during the ictal discharge. Similar testing should
also be performed in patients suspected of having nonepileptic
seizures, or pseudoseizures, because hyperventilation may
provoke a nonepileptic seizure in such patients.
Stimulus frequencies used
during intermittent photic stimulation range from 1 to 20 Hz in
increments of 2 to 3 Hz. In most subjects, a posteriorly
predominant, bisynchronous and timelocked "driving response" is
seen normally. The responses are best seen in the lower range
flash frequencies in the very young and in the midrange
frequencies in the adult. The absence of a driving response is
also normal. In some subjects, the driving response may appear
"spiky." Other normal findings during intermittent photic
stimulation include the electroretinogram (ERG), which is seen
in the frontopolar leads, and the photomyoclonic response (PMR),
which is a synchronous myoclonic response involving the
patient's facial and neck musculature, resulting in myogenic and kinesiogenic
artifacts on the EEG. The artifacts generated by the PMR may
appear as generalized spike or spike and wave activity. The PMR
must be differentiated from the photoparoxysmal response (PPR),
which is a burst of generalized epileptiform activity that is
evoked synchronously by the intermittent photic stimulation,
typically in the midrange frequency in susceptible patients, PPR may be seen in patients with an idiopathic generalized
epilepsy, such as juvenile myoclonic epilepsy or absence
epilepsy.
The process of becoming drowsy
(stage I sleep) and falling into deeper stages of sleep has been
shown to activate interictal epileptiform discharges of both
focal and generalized types. This is accomplished in the EEG
laboratory by recording during spontaneous sleep or sleep
induced by medications (e.g., chloral hydrate). Typically, the epileptiform discharges appear more frequently on a scalp EEG
during the lighter stages of sleep (stages I and II), and they
appear less frequently during deeper stages of NREM sleep
(stages III and IV) and during REM sleep. However, by using
depth electrodes in patients with intractable partial seizure
disorders, Rossi and colleagues demonstrated that interictal
epileptiform discharges increase in frequency with increasing
depth of NREM sleep.
The effect of sleep
deprivation is less well established. Although it is often used
as an activating method in patients with suspected seizures
after a routine EEG without epileptiform features, it is not
clear whether it causes any activation of the interictal
epileptiform activity beyond that caused by falling asleep.
Nevertheless, many EEG laboratories continue to recommend sleep
deprivation with sleep as a follow-up EEG after a nondiagnostic
routine EEG.
 |
Specific
Clinical Applications |
 |
Although
EEG is used most often as an ancillary test in clinical
epilepsy, it also is an invaluable tool in other neurological
conditions, such as encephalopathy, focal central nervous
system (CNS) lesions, and clinical brain death, as well as for electrocorticography, and in neonatal medicine. The following
sections discuss the usefulness of EEG in each of these
situations.
 |
Epilepsy |
 |
Epilepsy is defined as
"paroxysmal transient disturbances of brain function that may be
manifested as episodic impairment or loss of consciousness,
abnormal motor phenomena, psychic or sensory disturbances, or
perturbation of the autonomic nervous system. A seizure, or
ictus epilepticus, is an epileptic attack or recurrence. The
classification of epilepsies used by International League
Against Epilepsy (ILAE) includes two major categories: partial
epilepsies and generalized epilepsies. A partial seizure
disorder is considered to have a focal region of onset in the
brain, and awareness may be either preserved (simple partial
seizure) or lost (complex partial seizure). A generalized
seizure disorder is considered to involve most, if not all, of
the brain at onset. The generalized seizure types may involve
cessation of activity with loss of awareness (absence seizure)
or generalized tonic-clonic activity (generalized tonic-clonic
seizure). Both partial and generalized seizure disorders are
further subdivided into idiopathic and symptomatic types,
previously called primary and secondary, respectively.
Idiopathic epilepsies are thought to be genetically heritable,
are associated with normal intelligence, and occur during
specific age periods. The symptomatic epilepsies are likely the
result of a CNS injury, which in a symptomatic partial epilepsy
consists of a focal lesion and in a symptomatic generalized
epilepsy consists of diffuse cerebral abnormality. Symptomatic
epilepsies are typically lifelong conditions.
It cannot be overemphasized that the
diagnosis of epilepsy is based primarily on the clinical
history. As noted above, a clinical seizure rarely occurs during
an EEG, and thus the EEG is rarely diagnostic of a seizure
disorder or epilepsy. In a large, populationbased EEG
study by Zivin and Ajmone-Marsan involving subjects without a
history of seizures, approximately 2 percent of the subjects
had EEGs with epileptiform discharges. Of the individuals in
this subgroup, only
15
percent subsequently developed a seizure
disorder. Therefore, epileptiform discharges seen on an EEG
should not be referred to as interictal discharges unless it is
known that the patient has a clinically defined seizure
disorder. Focal or generalized epileptiform discharges should be
noted as consistent with the interictal expression of either a
partial or a generalized epilepsy, respectively. When applied in
the appropriate clinical setting, the EEG is useful in
classifying the seizure type, predicting the long-term outcome,
and choosing the appropriate antiepileptic medication.
Overall, symptomatic partial
seizure disorders are the most common type of epilepsy. The
clinical semiology of the partial seizure generally depends on
the site of onset. In children, focal epileptiform discharges
arising from the temporal region have the greatest incidence of
clinical seizures, ranging from 85 to 95 percent. The next
highest incidence (70 to 75 percent) is associated with frontal
discharges. The central, parietal and occipital regions have
the lowest incidence of seizures related to epileptiform
discharges. estimated at 40 to 70 percent. In addition to the
characteristics of recorded epileptiform activity, the age of
the patient and the presence or absence of neurological deficits
on examination are important factors that are helpful in
determining the clinical significance of epileptiform
discharges and in classifying the partial seizure disorder as
either symptomatic or idiopathic. The occurrence of a clinical
seizure with a focal electrographic correlate is diagnostic of
a partial epilepsy. Blume and colleagues presented several types of scalp EEG
correlates for partial seizures, most of which began with
rhythmic sinusoidal activity or repetitive sharp wave activity
that subsequently evolved in frequency. Most patients with
complex partial seizures were noted to have a scalp correlate on
the EEG. Patients with simple partial seizures were less likely
to have a scalp correlate.
The best-defined idiopathic
partial epilepsy is benign rolandic epilepsy. The classic EEG
finding in this childhood seizure disorder is a characteristic monomorphic centrotemporal sharp wave. The sharp waves are often
seen independently in the centrotemporal and adjacent regions,
and they are accentuated by light sleep. The waking
background rhythm is generally normal.
Of the idiopathic generalized
epilepsies, the absence seizure is the most common type. The interictal EEG feature of this type of seizure disorder consists
of generalized, high-amplitude, anteriorly predominant 3-Hz
spike and wave discharges, called
typical 3-Hz spike and wave. When the
spike and wave discharges occur repetitively, they are called
bursts. Although these discharges are called "3-Hz." the
initial frequency of the burst is 3 to 4 Hz, and the frequency
may slow to 2.5 Hz during more prolonged bursts. The discharges
are reactive to alerting maneuvers and may become fragmented in
deeper stages of sleep. Juvenile
myoclonic epilepsy (JME) is
another type of idiopathic generalized epilepsy. The spike and
wave discharges of this seizure disorder are also generalized
and anteriorly predominant, but they have an initial frequency
0f 4 to 6 Hz and may begin with a polyspike discharge. The EEG
of a patient with an idiopathic generalized epilepsy who is
maximally alerted is generally normal. During photic
stimulation, there may be a photoparoxysmal response in both
absence epilepsy and JME, which may be helpful in classifying
recognized epileptiform discharges as consistent with an
idiopathic generalized epilepsy rather than a symptomatic
partial or generalized epilepsy.
Epileptiform patterns in
symptomatic generalized epilepsies are of three types. A slow
spike and wave pattern at approximately
2
Hz is seen in patients with
mental retardation having multiple seizure types (atypical
absence, tonic, atonic, or tonic-clonic seizures), which is
known as the Lennox-Gastaut syndrome. A second type of interictal or ictal EEG pattern seen in patients with
symptomatic generalized epilepsy is generalized paroxysmal fast
activity (GPFA), which consists of bursts of rhythmic,
generalized beta activity. When the bursts are seen during
wakefulness, they are commonly accompanied by a tonic seizure.
During sleep. bursts of GPFA not accompanied by clinical changes
are considered an interictal pattern. The third pattern of
epileptiform activity in secondary generalized epilepsy is an
atypical generalized spike and wave pattern, consisting of
generalized 3 to 6-Hz spike or polyspike and wave activity. The
waking background in patients with secondary generalized
epilepsies is abnormally slow, including slowing of the
posterior background rhythm and generalized slowing.
In patients
suspected of having a seizure disorder, a normal routine, awake
EEG should be followed with either a natural or
medication-induced sleep EEG or a sleep-deprived EEG. Before the
advent of long-term video-EEG monitoring for the diagnosis of
possible seizures, three or more EEGs were often obtained to
confidently conclude normality and absence of epileptiform
activity. Because antiepileptic medications have been shown not
to affect the frequency of focal interictal epileptiform discharges, the
decision to treat a patient for a suspected partial seizure
disorder should not be based solely on the initial EEG findings.
Conversely, the EEG has not proven to be a reliable tool in
predicting whether a patient's antiepileptic medication can be
discontinued. In patients with an idiopathic
generalized epilepsy, treatment with
appropriate antiepileptic
medication may eliminate all interictal epileptiform activity on
the EEG. Therefore, the decision to discontinue an
antiepileptic medication in a patient with a seizure disorder
should be based on the type, etiology and response to
medications of the seizures and not on interictal EEG findings.
 |
Encephalopathy
and Coma |
 |
Encephalopathy and coma result from conditions that
affect both cerebral hemispheres or the reticular
activating system in the midbrain. The differential
diagnosis is broad, including metabolic, toxic,
anoxic/ischemic, infectious, endocrinologic, degenerative, and inflammatory
processes. These processes affect the brain diffusely, and,
consequently, changes in the EEG often appear generalized.
While most EEG findings in encephalopathy and coma are
nonspecific with regard to etiology, information relevant to the
clinical course and prognosis can be obtained using the EEG.
In cases of mild
encephalopathy, theta and delta activity is intermixed with the
background alpha rhythm. Occasional generalized delta
transients are also seen. As the encephalopathy worsens, there
is loss of background alpha-range frequencies and an increased
amount of generalized theta and delta activity.
Intermittent-rhythm delta activity (IRDA) may appear, which in
adults generally is frontally predominant (FIRDA), and is
consistent with moderate diffuse bihemispheric cerebral
dysfunction (Fig-6).
In
severe encephalopathy, there is generalized delta
activity. Loss of reactivity in anyone of these stages implies
greater severity, and, in specific clinical settings, a worse
prognosis. In the clinical setting of severe anoxia (e.g.,
after cardiac arrest) or severe closed head injury, invariant
patterns of persistent, generalized alpha activity (alpha
coma), generalized periodic epileptiform discharges, or the
burst suppression pattern (Fig-7) are associated with very
poor outcome.
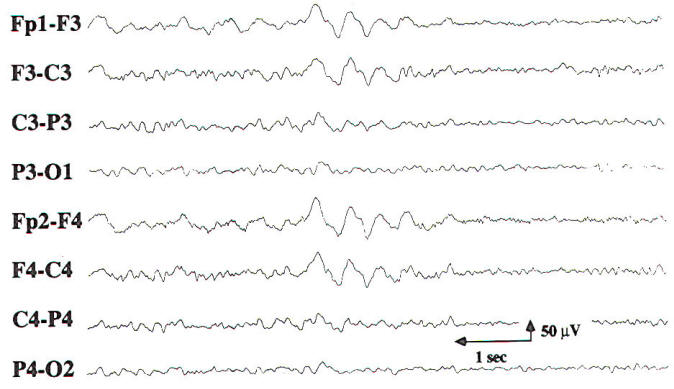 |
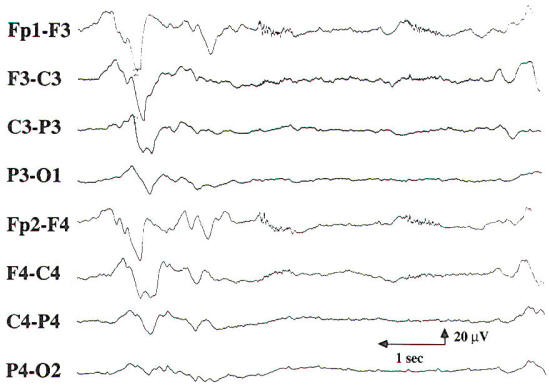 |
Fig-6: Frontal intermittent
rhythmic delta delta activity (FIRDA) |
Fig-7: Burst suppression pattern,
consisting of bursts with an initial delta transient
and superimposed theta activity lasting 2 s. During
the burst intervals, there is no EEG activity.
Ventilator artifacts are seen. |
In the
early reports of the EEG findings in hepatic coma, triphasic
waves were noted which were initially thought to be
pathognomonic for this condition. These three-phased
generalized discharges consist of high-amplitude, sharp wave
complexes that are repetitive, have an average frequency of 2
Hz, and show initial surface positivity and anterior
predominance (Fig-8).
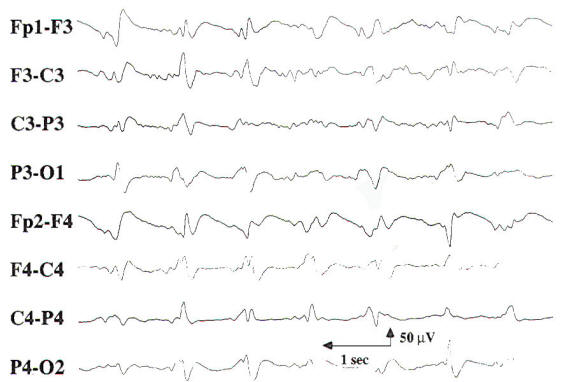 |
|
Fig-8: Periodic triphasic waves at
1-Hz frequency. |
|
Triphasic waves may be intermittent and reactive,
or they may be persistent and unreactive. There is no normal
background rhythm. Although present on the EEG in most patients
with hepatic failure, triphasic waves may also be seen in cases
of other metabolic, toxic, anoxic, degenerative and inflammatory
encephalopathies. In patients whose EEGs demonstrate triphasic
waves, overall mortality is high, and there are few normal
survivors. Periodic sharp waves having a morphology similar to
that of triphasic waves may be seen in patients with
Creutzfeldt-Jakob disease (CJD), but the frequency of the
discharges typically averages 1 Hz. In early CJD, the periodic
complexes are superimposed on a background that may have only
mild slowing. As the disease progresses, the background rhythm
is lost, resulting in a pattern of periodic 1-Hz discharges on a
flat background. The clinical history of subacute dementia,
seizures, and myoclonus in conjunction with this periodic
pattern is strongly suggestive of CJD. To confirm this
progression, sequential EEGs may need to be performed during
the course of CJD.
Epileptiform activity may be
seen on the EEG in some degenerative encephalopathies that have
associated seizures. Multifocal, independent epileptiform spike
discharges may be seen in TaySachs disease, in several of the
progressive myoclonic epilepsies (neuronal ceroid lipofuscinosis,
Lafora body disease, and some mitochondrial encephalomyopathies),
and in Rett syndrome. Atypical generalized spike and wave
activity is present in UnverrichtLundborg disease, which is
another type of progressive myoclonic epilepsy.
Of the inflammatory
encephalopathies, distinctive EEG findings are seen in subacute
sclerosing panencephalitis (SSPE) and herpes simplex
encephalitis. The clinical presentation of SSPE includes
myoclonus with progressive encephalopathy. The EEG shows
periodic, polyphasic sharp and slow wave complexes that have an interburst interval of 4 to
10 s. As SSPE progresses, there is
gradual loss of the intermixed background frequencies, resulting
in a pattern similar to burst suppression. Herpes simplex
encephalitis is the most common sporadic viral encephalitis,
typically presenting with fever, encephalopathy, and
secondarily generalized seizures. The EEG commonly shows
periodic lateralized epileptiform discharges (PLEDS). which are
lateralized to the side of the herpes infection. Should both
temporal lobes be involved, bilateral independent periodic epileptiform discharges (BIPLEDS) may be seen on the EEG. Other
forms of inflammatory encephalopathy typically result in
nonspecific slowing of the EEG, the severity of which is often
correlated with the severity of the encephalopathy.
Lastly, nonconvulsive status
epilepticus should be considered in patients with a known
seizure disorder or recently witnessed seizure who present with
prolonged encephalopathy. Patients presenting in nonconvulsive
status epilepticus may have subtle clinical findings of ongoing
seizures, and electroencephalography is crucial in confirming
response to therapy with cessation of electrographic seizure
activity. The EEG in nonconvulsive status epilepticus generally
shows widespread, repetitive sharp and slow wave complexes at 1
to 2 Hz. Administration of low-dose intravenous benzodiazepine
therapy during the EEG usually results in rapid resolution of
the ictal pattern and clinical encephalopathy. Should convulsive
seizure activity not respond to conventional therapeutic
intervention, then barbiturate coma or general anesthesia with
concurrent EEG monitoring is needed to demonstrate a burst
suppression pattern and lack of electrographic seizure activity.
 |
Focal Lesions
of the Central Nervous System |
 |
The EEG findings in focal
cerebral lesions are generally nonspecific. Serial EEGs may be
necessary to fully appreciate the electrographic changes in
conditions where there may be significant change in neurological
status, such as acute stroke or progressive brain tumor. If only
the cortical gray matter is involved, there is amplitude
suppression of the surrounding EEG activity. However, many focal
cerebral lesions involve both the cortical gray matter and the
underlying white matter, resulting in slowing of the EEG
activity with intermittent focal delta activity. Midline and
infratentorial lesions may not produce any changes in the EEG,
or they may result in generalized slowing.
When focal delta activity is
intermittent, it is consistent with focal cerebral dysfunction
of a nonspecific etiology. Focal delta activity that is nonreactive and is present for 70 to 80 percent of the record
(Fig-9) is called persistent polymorphic delta activity (PPDA).
PPDA is a specific finding in structural lesions of the brain,
often seen in patients with a supratentorial high-grade
cerebral neoplasm, a large cerebral abscess, or a stroke
involving subcortical and cortical regions. Transient PPDA may
be seen after a complicated migraine headache or a partial
seizure. emphasizing the need for serial EEGs in certain cases.
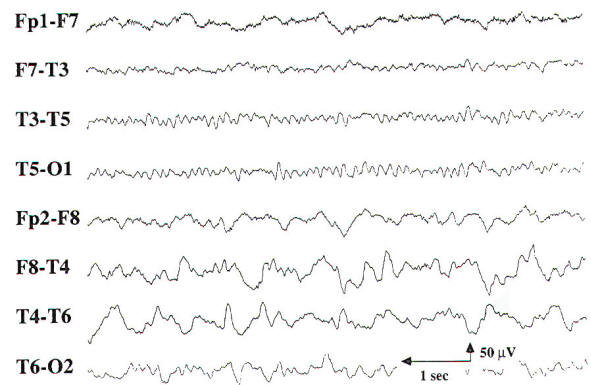 |
|
Fig-9: Persistent polymorphic
delta activity (PPDA) in the right temporal region. |
|
As
discussed above, intermittent rhythmic delta activity (lRDA) is
generally a finding consistent with diffuse bihemispheric
cerebral dysfunction. However, in a large series of patients
with IRDA, brain tumor was seen in 30 percent and cerebrovascular disease in 19 percent. Although reported
before the advent of computed tomography (CT), the
diagnoses in this study were based on neuropathologic
confirmation. The frontal lobe is the most common location for
brain tumors associated with IRDA on the EEG.
Sharp waves or spike
discharges are occasionally seen on the EEGs of patients with
focal cerebral lesions. The epileptiform discharges are rarely
the sole abnormality on the EEG, and they are most often
associated with focal delta slowing. Periodic lateralized epileptiform discharges (PLEDs) may be seen in acute cerebral
lesions such as stroke or herpes simplex encephalitis. PLEDs may
be unilateral or bilaterally independent, termed BIPLEDs (Fig-10). PLEDs are generally self-limited, lasting
1 to 2 weeks
during the acute phase of illness. There is a high incidence of
seizures in patients whose EEG demonstrates PLEDs or BIPLEDs
(Fig-11). Last, patients with a focal cerebral lesion may
present in partial or generalized status epilepticus.
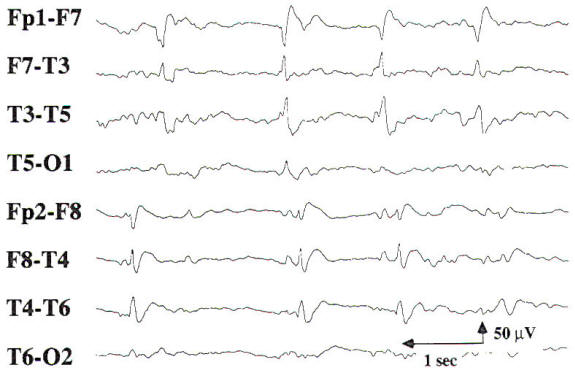 |
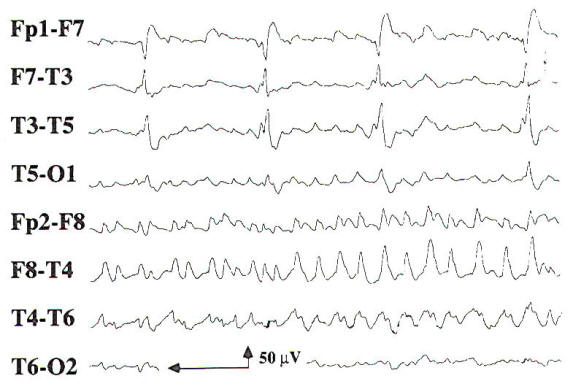 |
Fig-10: Bilateral independent
periodic lateralized epileptiform discharges
(BIPLEDs) due to right ICH and IVH. |
Fig-11: Focal seizure in right
temporal region with ongoing PLEDs in the left
temporal region. |
 |
Brain Death |
 |
Brain death
has been defined as the "irreversible cessation of all
functions of the entire brain, including the brainstem." The
determination of brain death is important in clinical
situations such as potential organ donation and withdrawal of
life support. The clinical criteria for brain death in adult
patients can be summarized as follows:
1.
There is no known
reversible etiology. Reversible factors that may cause coma or
apparent coma must be ruled out, including sedative medications
and paralytics (e.g., barbiturates, benzodiazepines,
neuromuscular blocking agents), hypothermia (i.e., the core
temperature must be greater than 32.2°C), a potentially
reversible medical illness (e.g.. hepatic failure, renal
failure), and shock.
2.
Coma and the absence of
brain stem function (e.g., cranial nerve function and
respiratory control) are demonstrated by a neurologist or
neurosurgeon on two successive neurological examinations
separated by an appropriate period. In adult patients, 12 h is
generally an adequate period between examinations. However, in
adults with anoxic/ischemic encephalopathy and in children, this
interval may extend to 24 h or longer, depending on the
circumstances. Criteria for newborns are not well established.
3.
Confirmatory tests (e.g., EEG, cerebral angiogram or nuclear
cerebral blood flow scan) may be used if the period of
observation is less than that recommended above, as in the
setting of organ donation. In all other circumstances, these
tests are considered optional and are used at the discretion of
the attending physician.
An EEG
recording to determine brain death should not be considered
until the clinical criteria are met. The EEG then be ordered to confirm
electrocerebral inactivity or silence. (ECI and ECS.
respectively). ECI is defined as lack of EEG activity greater
than 2 µV. The following guidelines for performing an EEG to
confirm ECI have been recommended by the American
Electroencephalographic Society:
1.
At least 8 scalp
electrodes should be used, covering the frontal, central,
temporal, and occipital regions of both hemispheres.
2.
Interelectrode impedances
should be between 100 and 1000 Ω.
3.
The integrity of the
recording system should be confirmed at the beginning of the
recording. This is generally done by touching each electrode in
succession and documenting the resulting electrode artifact.
4.
Interelectrode distances
should be 10 cm or greater.
5.
Sensitivities must be
increased from 7
µV /mm to 2
µV /mm during the recording, the
duration of which should be at least 30 min, excluding time for
EEG machine preparation (i.e., machine calibration at all
sensitivities).
6.
Filter settings should
be 1 Hz for the low-frequency filter and 30 Hz or greater for
the high-frequency filter.
7.
Monitoring of additional
cerebral and noncerebral sites should be done as needed. This is
done to confirm the source of suspected artifacts, such as
electrocardiogram, respiration. electromyogram. etc.
8. Unreactivity of the EEG
should be documented using visual stimulation, auditory
stimulation and somatosensory stimulation below and above the
neck.
9.
The EEG recording during ECI should be performed by a qualified technologist.
10.
The EEG should be
repeated if there is any doubt regarding the diagnosis of ECI.
It is crucial to remember that
the EEG is only a confirmatory test for the presence of cerebral
death and that the primary criteria are clinical. As the EEG is
subject to artifacts whose source may not be determined, the
utility of this test for confirmation of ECI may be limited in
settings where factors which cause EEG artifacts are prevalent.
 |
Electrocorticography |
 |
Electrocorticography (ECoG) is
the technique by which the brain's electrocerebral activity is
directly measured using either depth electrodes, cortical surface
contact electrodes, or subdural electrode strips or arrays.
Although ECoG is not a routine procedure, it has become widely
used in the presurgical evaluation of patients with medically
intractable partial epilepsy where the site of seizure onset
cannot be adequately localized using noninvasive methods. ECoG
has also proved to be an important technique for functional
brain mapping of eloquent cortex during the neurosurgical
resection of lesions such as brain tumors or vascular
malformations.
In patients undergoing
invasive monitoring with depth or subdural electrodes for
epilepsy surgery evaluation, the decision of where to place the
electrodes is based on several factors. including ictal
semiology, interictal epileptiform activity and neuroimaging
findings. Electrodes should be placed to cover the region of
suspected seizure onset. Often the corresponding contralateral
cortex is also covered, for reference and to confirm that there
is a single zone of epileptogenesis. A sufficient number of
seizures are recorded with video-EEG monitoring using the
invasive electrodes, and the behavioral onset of seizures is
timed to confirm that it follows the electrographic onset. Interictal epileptiform activity is much more often recorded
when invasive electrodes are used, and it is often multifocal.
Upon completion of monitoring, depth electrodes may be removed
at the patient's bedside. Removal of subdural electrodes is
generally performed in the operating room.
The primary
use of cortical stimulation is to identify areas of essential
cortex, such as those subserving motor, sensory or language function.
Brain mapping using cortical stimulation may be performed during
epilepsy surgery or other neurosurgical procedures such as the
resection of tumors or vascular malformations. It may be
conducted intraoperatively with the patient awake in the
operating room, or extraoperatively in the patient's room, where
testing may be performed over several days in sessions lasting 1
to 3 h as needed.
At most centers, stimulation consists of 0.3 to 1
ms biphasic square wave pulses at 50 Hz, lasting from 2 to 5 s
each. The stimulation intensity starts at 0.5 to 1 mA and is
raised in increments of 0.5 to 1 mA until a neurological deficit
is produced or afterdischarges occur or a maximum 15 mA stimulus
intensity is reached. During dominant temporal lobe surgery,
object naming alone may be used if the zone of resection is more
than 2 cm distal to the defined language cortex. However, when
the zone of resection must border on language cortex, more
extensive testing is performed, including reading, repetition,
naming and comprehension. Motor and sensory areas may be
similarly mapped by evoking either muscle
contraction when stimulating areas of the precentral gyrus or
regions of paresthesia when stimulating the postcentral gyrus.
In all cases, the lowest stimulation intensity that evokes a
response should be used, to limit the current field to the
region of interest.
Clinical seizures provoked by
cortical stimulation are in general not predictive of the zone
of epileptogenesis in patients with intractable partial
seizures. Afterdischarge potentials are brief, self-limited
electrographic seizures that may be produced by cortical
stimulation. Afterdischarges may evolve into a clinical seizure,
and for this reason, anticonvulsant levels in patients with
partial seizures are maintained in the therapeutic range when
cortical stimulation is being performed. At some medical
centers, cortical stimulation is performed in an attempt to
induce typical auras that the patient may experience. Temporal
lobe epilepsy has been reported to have the highest concordance
between spontaneous seizures and induced auras or seizures.
 |
Neonatal EEG |
 |
The neonatal period extends
from birth (including premature birth) to age 2 months. The
conceptual age (CA), or age since conception, is an important
factor in interpreting the neonatal EEG, because it defines the
level of maturation of the CNS. As the premature brain matures,
well-defined patterns are seen that help to differentiate normal
from abnormal EEGs at specific ages. Owing to the small size
of the neonatal head, the International
10-20
System of electrode placements
is modified to allow for coverage of the frontal, central,
temporal, and occipital regions. Physiologic parameters such as
heart rate, respirations, eye movements and limb myogenic
activity are also monitored to help differentiate active sleep
(rapid eye movements, variable heart rate and respiration) from
quiet sleep (no movement with regular heart rate and
respirations), and wakefulness from sleep. The EEG is performed
at a paper speed of 15 mm/s (one-half the adult paper speed).
This is done to allow a longer sampling time (generally 1 h) and
to compress the EEG, as it consists predominantly of theta and
delta range frequencies. Above a CA of 48 weeks, the standard
EEG is performed.
Before 22 weeks CA there is no
discernible electrocerebral activity. As the neonatal brain
matures, a discontinuous, invariant pattern is seen initially,
which is gradually replaced by more continuous, variant
patterns as term gestation is reached. At 26 weeks CA, a
discontinuous pattern is seen, with bursts of high-amplitude,
sharply contoured theta activity, which is maximal in the
temporal regions. During the interburst periods, there is no
discernible EEG activity. The EEG is unreactive and remains so
until 34 weeks CA. By 30 weeks CA, active sleep can be
distinguished from quiet sleep by a reduction in amplitude and
the amount of delta activity. At this age, beta-delta complexes
(delta brushes) are seen in both stages of sleep; they gradually
become less frequent and disappear by term. By 35 weeks CA, the
EEG is reactive. Independent frontal sharp transients are seen,
as well as occasional equally distributed independent sharp
transients in both hemispheres, which may be seen until term.
Wakefulness can be distinguished from sleep at 36 weeks CA,
demonstrating continuous, low-amplitude mixed frequencies. At
term, the EEG should be synchronous and reactive and should
demonstrate both active and quiet sleep and wakefulness.
The most common abnormality in
a neonatal EEG is the absence or delayed appearance of normal
patterns. A low-amplitude EEG may be due either to cerebral
dysfunction or to an extracerebral fluid collection such as
scalp edema or a subdural hematoma. An increased number of
multifocal independent sharp transients indicates diffuse
cerebral dysfunction, which is maximal in the region of the most
frequent transients. These sharp transients are not called sharp
waves or spikes, as they do not indicate a seizure disorder in
the neonate.
The clinical and EEG findings
of a seizure in the neonate vary in semiology and pattern, and
some are controversial. Seizures in a neonate may not be
accompanied by an electrographic correlate. Conversely,
electrographic seizures may have no clinical correlate, or they
may have subtle correlates such as apnea or heart rate changes.
The EEG diagnosis of a seizure is based on the evolution of
frequency of focal rhythmic activity, which may be limited to a
single electrode. Generalized tonic clonic seizures very rarely
are seen, likely because the myelination and dendritic
arborization of the CNS is limited at this age.
Obtaining
an EEG should be considered in all premature or term neonates
who have evidence of significant neurological dysfunction on
clinical examination. In addition to providing information
regarding CNS maturation, the neonatal EEG is often helpful in
guiding neuroimaging assessment by cranial ultrasound, CT, or
MRI. As neonatal seizures may have subtle or no clinical
manifestations, the EEG is an invaluable tool in the clinical
evaluation of the neonate.
|
 |
Starting from July-2007 all the surgical activities of
Prof. Munir Elias will be guided under the electrophysiologic control of
ISIS- IOM
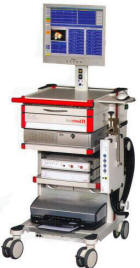
ISIS-IOM Inomed Highline

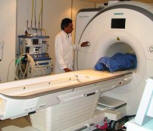
Starting from 28-November-2013 Skyra with all clinical applications in
the run. |
|